Gas separation processes have a great impact on the current needs of human society including medical, environmental, energy and high-value chemicals necessities. Some of the most popular applications for these gas processes are CO2 capture, natural gas sweetening, O2 purification, hydrogen recovery, and noble gas separation. When talking about gas separation, zeolite-based materials may pop-up in your mind. No wonder, they have been used for this purpose over the last four decades. But newer materials could outperform them.
In the past, high-energy consuming processes like cryogenic distillation were used to separate gas mixtures. When zeolite-based materials came out to the market, they offered similar or better results with less power consumption making the process cheaper (from medium to small-scale processes, cryogenic distillation is still used at large scale). Porous solid adsorbents like zeolites are materials with high surface areas, increasing the available active sites for chemical interactions. Since zeolites have been studied for several decades, a variety of synthesis techniques made the chemistry tunable for specific applications increasing selectivity. The main mechanisms of action associated with these materials are size separation (zeolites are also known as molecular sieves) and chemical affinity exclusion (the compound of interest is retained in the packed bed).
Zeolites are commonly used as powders and membranes in pressure swing adsorption (PSA) and membrane separation technologies. The use of zeolites in PSA processes allows separating the gas mixtures at ambient temperature. This technique decreases the energy consumption to only 10% of the energy required by conventional distillation processes. On the other hand, its implementation in membrane separation technology aims to more selective separations, although membranes processes are theoretically suitable for scale-up, they still had limitations due to its low thermal and chemical stability in a wide range of operation conditions.
Alternative adsorbent materials for gas separation
Over the past two decades, Metal-Organic Frameworks (MOFs) emerged as an alternative to conventional porous materials (like zeolite, silicates, and activated carbon). MOFs offer higher surface areas than zeolites. Actually, to this date, metal-organic frameworks are the most porous materials known to have specific surface areas as high as 7,140 m2/g (structural changes of the same material predict a higher value of 10,000 m2/g). Unlike zeolites, MOFs are materials consisting of structures formed by metal nodes bonded by organic ligands. This allows many combinations, 88,000 structures are already reported and the possibilities for creating new structures are higher, theoretical studies say hundreds of thousands of MOFs are possible.
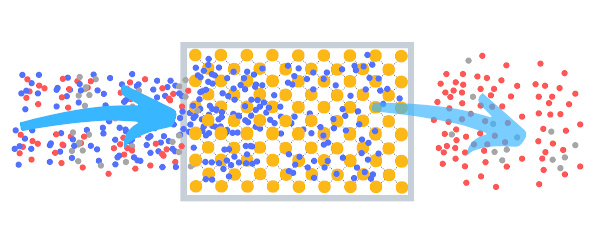
Molecular sieve clean the gas stream from undesired or desired molecules by adsorption, molecular sieving effect or Knudsen effect. The above picture shows the adsorption of certain molecules in a adsorption filter.
All the combinations of different metals and organic molecules increase the ability to tune-up the size and structures of the pores. For example, the size of the pores can be manipulated by changing the length of the organic molecule used to coordinate the metal ion (the pore size can range from 3 to 100 Å). In the same way as pore size manipulation, chemical tailoring is possible by using metal nodes and organic molecules of different nature. This powerful tool allows making gas separation considering other properties like the polarity, coordination abilities, and conformations, improving the selectivity of a given material towards specific products. An example of these advantages can be found in its implementation in high-efficiency oxygen concentrators.
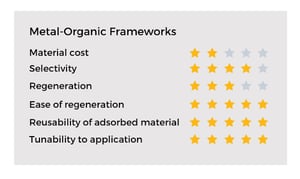
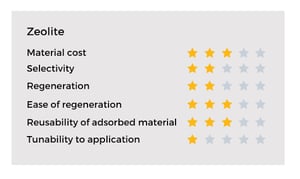
Similar to zeolite materials, MOFs offer effective processes and even require less amount of material due to the high specific surface area. These novel materials have found multiple applications such as heterogeneous catalysis, electric devices, water purification, and gas adsorption and separation. However, these systems are limited to small and medium scale to make the process feasible in terms of material costs.
MOF-5 showed twice the CO2 adsorption capacity of zeolites
Regarding specific applications, an example of the advantages of MOFs is that they have a more selective CO2 adsorption over zeolites in post-combustion streams, the high selectivity is achieved through the manipulation of the pore size and shape in MOFs, i.e. MOF-5 showed a higher CO2 adsorption capacity of 50 wt% compared to the 25.1 wt% in zeolites. The presence of cations such as Na(I), Ca(II), or Li(I) in zeolites affect the adsorption capacity because of the interaction between alkali cations and CO2 molecules.
Conclusions
Metal-Organic frameworks offer the same advantages as other nanoporous solid materials for gas adsorption and separation like cost-effective processes. But they are outstanding in other aspects such as the ability of size and structure manipulation of the pores as well as easy chemical reactivity tailoring, increasing capacity and selectivity of the materials. The implementation of MOFs may add further cost-saving strategies since less material is needed to process the same amount of gas stream. If you have a gas separation process, you should consider taking a look at the alternatives and advantages offered by MOFs.