The specific characteristics of inert gases – e.g. they are almost non-reactive and non-flammable – make them perfect for application in lighting, welding, medical applications or diving gear. However, given their limited abundance in the atmosphere and their high production cost, their application is restricted to certain fields. While we can’t change the occurrence of noble gases, production cost could potentially be optimised.
Helium, Neon, Argon, Krypton and Xenon are used in many applications nowadays; from creating inert atmospheres, leak detection and lighting to fire extinguishers, medical devices and food preservatives. The main interest in noble gases are the inert properties they provide in high purity. This also means, that most applications require high levels of purity. Only Radon, a naturally occurring radioactive gas, has almost no application today as it has been replaced in many previous uses by safer and cheaper alternative measures.
Low abundancy – high purification cost
Natural abundancy of inert gases range from 5.2 ppm (Helium) to 0.086 ppm (Xenon) in the earth’s atmosphere. Such low concentrations make it hard to purify the gases for industrial applications. In almost all cases, fractional distillation is the only option to achieve the required purity.
Fractional distillation is based on different boiling points of the gases in a gas mixture. As the gases in the mixture will change their phase at different temperatures, the desired gas can be separated. In the case of Argon, the gas mixture needs to be cooled to or below −185.848°C in multiple stages. However, cooling the gas mixture to such low temperature is very energy intensive. This is directly reflected in the prices of each noble gas.
Adsorption instead of fractional distillation
To circumvent the high costs of noble gas purification with fractional distillation, pressure or temperature swing adsorption with advanced materials could prove to be efficient and less energy intensive. Commonly used adsorption materials are activated carbon and zeolites. However, they show low selectivity and adsorption capacity. Furthermore, activated carbon reacts with NOx (which is present in certain industrial gas mixtures such as off-gas) – a fire hazard that is best avoided.
Another potential candidate for gas separations are metal-organic frameworks (in short MOFs) – crystalline compounds of metal ions and organic linkers that form hollow scaffoldings. These materials can be tailored to the specific applications by combining metal ions and linkers accordingly. Therefore, they promise to offer great value in various industries – including different gas separations.
Helium, Neon and Argon – well established solutions but some alternatives available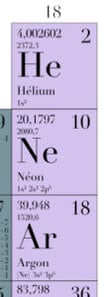
There are alternative approaches to fractional distillation of Helium, Neon and Argon based on activated carbon or zeolite, but research is rather limited.
For example, using pressure swing adsorption on activated carbon purifies Helium from natural gas with 0.06% in the gas mixture to more than 99% purity in laboratory setup. In contrast, cryogenic distillation is economically viable only above 4% Helium in the natural gas mixture. Activated carbon can also adsorb trace amounts out of gas streams to purify Neon. Similarly, adsorption of contaminants seems to be the more expedient solution rather than pressure or temperature swing adsorption of Argon itself.
The use of metal-organic frameworks in mixed matrix membranes for the separation of noble gases could improve their selectivity. Matrimid membranes were ugraded with the MOFs HKUST-1 and Cu-BDC to separate Helium from Methane and Nitrogen. The selectivity of the membranes has increased by more than 96% membranes to 257.9 (He/CH4) and 193.4 (He /N2) compared to standard Matrimid.
Given the tuneability of MOFs, there is still room for further development in adsorption purification of these gases.
Krypton & Xenon – high value meets high selectivity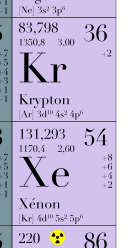
Krypton is used in energy-saving fluorescent lights and after reaction with fluorine gas to krypton fluoride in certain lasers. Cryogenic distillation of air is used to produce Krypton, but it could also be capture out of off-gas streams from nuclear reactors. Xenon is used in specialized lighting, ion propulsion systems in satellites, etching of silicon microprocessors and in medical applications. Given its second lowest abundancy of all inert gases (0.086 ppm), it’s also the most valuable at around 40 $/l.
While both noble gases are adsorbed onto different adsorption materials, most have a higher selectivity towards Krypton rather than Xenon. NaX and NaA zeolites only show low Xenon uptake at room temperature but higher selectivity than activated carbon. As activated carbon reacts with NOx (present in natural gas mixtures) to explosive gases, it won’t see any commercial application. However, doping zeolites with silver nanoparticles can increase the Xenon uptake.
Multiple metal-organic frameworks including the well-known HKUST-1 and MOF-74 show great uptake (up to 6.1 mmol/g) and selectivity (up to 22) at standard conditions. Xenon is adsorbed at open metal sites on the surface of the materials. Depending on the pore size and the MOF, most of the adsorption takes place on the outer surface of the MOF rather than within the material. A lot of research has been done using HKUST-1 with very promising results at standard conditions as well as at higher pressure/temperature or even in vacuum reactors. Other potential MOFs are PCN-12, MOF-74 (Ni), MOF-505, SIFSIX-3, UiO-66, ZIF-8 and NOTT-100.
On the other hand, small-pore MOFs can be used to separate Xenon selectively according to molecule size. This could offer molecular sieving opportunities that could further apply for other gases.
A study evaluated the separation of Krypton and Xenon from off-gas streams by using six different MOFs in the adsorption bed. Unfortunately, their findings weren’t tested in an experimental setup.
Radon – additional capture methods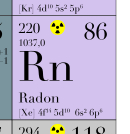
The radioactive noble gas Radon has almost no commercial application nowadays, but is considered a health hazard. Radon is a product of the natural decay of radium-226 – which is found in various rocks. As it is thus emitted from the ground in differing concentration, it can lead to high concentrations in buildings and especially mines.
Usually, isolation of the source and good ventilation will solve hazards of larger concentrations of Radon. Metal-organic frameworks have been evaluated to limited extend for the capture of Radon. However, various functionalized IRMOF-2, especially IRMOF-2 (I), have shown selectivity for Radon over Nitrogen in computational separation simulations. Furthermore, the MOF ZIF-12 shows even better selectivity towards Radon over 02 at standard conditions (Rn/N2 selectivity of 1800). Its selectivity even increases with decreasing amounts of Radon in the gas mixture. ZIF-12 could thus be a potential candidate for indoor Radon capture.
Conclusion
The industrial purification of noble gases in cryogenic distillation has significant drawbacks due to the large energy consumption during the process. Using adsorptive materials could improve the process (cryogenic adsorption) or even replace the cooling completely.
Metal-organic frameworks are high potential candidates especially for the least abundant noble gases such as Krypton and Xenon. As MOFs are highly selective, the purification process could be tailored towards the gas mixture from various sources such as natural gas or direct air capture. At this stage, first mover companies could greatly advance their position in the market by testing the materials on a pilot scale.